Ever found yourself facing data that doesn’t quite add up? Perhaps your activity assay is positive, but yourSDS-PAGE gel doesn’t have a matching protein band.
Or maybe you have limited options for detecting your molecule of interest because you don’t have an antibody.
Mass spectrometry (mass spec, or MS) could be the answer. It lets you identify molecules that are present at extremely low concentrations in your sample that may not be detectable by any other method.
It works by breaking up your molecule of interest into ion fragments—and there are many ways to do this, each suited to different types of molecules.
This article briefly explains the different ionization methods in mass spec and gives you some guidance picking the best one for your analyte.
What Is Mass Spectrometry? A Brief Recap
You might be wondering—a technique that measures mass! Isn’t that the job of a chemical balance? Yep. But measuring the mass of individual molecules is complex and requires precision, which mass spectrometry provides.
Mass spec has been a fundamental part of research for nearly a hundred years. The utility of mass spectrometers expanded after the 1990s with the discovery of various ionization methods (which we’ll discuss later), that enabled the detection of increasingly large molecules, such as macromolecules like proteins, nucleic acids, and protein–ligand complexes.
With evolving technological advancements and more sophisticated protocols, mass spectroscopy has become an indispensable tool for biological research and drug development programs. [1]
It can help address the following research areas:
- Positive assay results but no corresponding protein band on gels: When an activity assay indicates protein presence, but SDS-PAGE gels reveal no band, mass spec can confirm or identify the protein.
- Unidentified molecular signals: If a molecular signal lacks a clear identity, mass spec can provide structural data to pinpoint the compound.
- Limited detection methods due to antibody availability: When specific antibodies are unavailable, mass spec offers a viable alternative for detecting and characterizing target molecules.
- Confirming purity or heterogeneity: Mass spec can determine sample purity by distinguishing between the target molecule and potential contaminants or degradation products.
- Tracking post-translational modifications: Mass spec can detect and quantify modifications like phosphorylation or glycosylation that influence protein function.
- Quantifying low-abundance proteins: For proteins expressed at low levels, mass spec can provide the sensitivity needed to identify and quantify these targets.
Charge, Deflect, and Detect. How Mass Spec Works
Before diving into the different ionization methods in mass spec, it’s worth recapping how it works.
Mass spectrometry is an analytical technique used to accurately measure the mass and identify molecules based on their mass-to-charge (m/z) ratio. This Bitesize Bio article gives a brief end-to-end explanation of mass spec for biological research.
The sample to be analyzed is first injected into an HPLC liquid chromatograph for separation and purification based on its physicochemical properties, which determine its affinity for the column being used.
The separated molecules then proceed to mass spectrometry where the molecules are ionized, splitting them into charged fragments, and separated based on their mass-to-charge (m/z) ratios. Since the purification through HPLC and the analysis by MS occur together, this technique is often referred to as LC-MS.
The resulting spectrum displays m/z values on the X-axis and the detected intensity of the different fragment ions as peaks on the Y-axis. This spectrum is effectively a “fingerprint” of the analyte. That’s to say, it’s unique to each molecule. Check out Figure 1 below for an example spectrum.
Like many analytical methods, the intensity of the signal is related to the abundance of that particular ion.
By deconvoluting ions with similar masses but different charges, or different masses with the same charge, we can determine the exact mass of the species present in the sample, revealing both:
- identity (mass)
- and heterogeneity (purity) of the sample
And by definition, positively identifying your analyte using its m/z ratio indicates its presence (anything other than a non-zero value).
Though technology has advanced significantly, the basic principles remain the same. A typical mass spectrometer consists of three main components:
1. Ion Source
Ion source, as the name suggests, ionizes the analyte. Since the separation of the molecules in mass-spec occurs based on the mass-to -charge ratio, ionization is essential. [2]
Molecules are ionized by adding or removing electrons. These charged molecules are called molecular ions.
Based on the intensity of the energy required during the ionization process, ionization could be a hard or soft ionization.
- Hard ionization imparts a relatively large amount of energy, causing significant analyte fragmentation by disrupting covalent bonds. Hard ionization utilizes atoms such as Xenon or Argon to facilitate the ionization of the analyte.
- Soft ionization imparts less energy, resulting in minimal fragmentation of the analyte, and is accomplished through chemical ionization of analytes using reagent gases such as methane or ammonia.
2. Mass Analyzer
A mass analyzer is a vacuum-sealed compartment. Here, the ionized molecules are induced to move or “fly” under the influence of an electric or magnetic field.
The flight paths of the charged molecules will vary based on their mass-to-charge ratio which is measured by a detector. [2]
Different types of mass analyzers include:
- Quadrupole
- Ion Trap
- Orbitrap
- Time of Flight (ToF)
3. Detector
A detector, the final component of a mass spectrometer, measures the m/z ratio and intensity of each ion after separation. [3]
Types of detectors include:
- Electron multipliers
- Faraday cups
- Ion-to-Photon detectors
As each ion hits the detector, a peak is added to the spectrum with its m/z value on the X-axis and intensity on the Y-axis. [2]
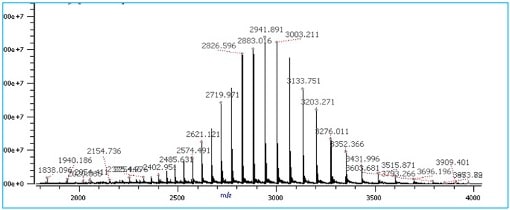
Figure 1. A typical mass spectra of a purified protein molecule where each individual peak has a different m/z ratio are differently charged molecular ions generated during ionization of the analyte. The analyte used to generate the MS spectra was serratiopeptidase. (Image credit: Vishal Srivastava.)
Making them Fly. Ionization Methods in Mass Spec
Creating ions is crucial for separating and identifying molecules in mass spectrometry. There are several ionization methods, which I’ll explain in detail.
Electron Impact Ionization (EI): Hard ionization
This method is the preferred choice for ionization of small (<600Da), non-polar, volatile organic analytes.
As the name suggests, the technique uses the impact of electrons with the sample to ionize the analyte molecule.
First, the sample is volatized (rapid conversion to a gaseous state) and introduced into the system under vacuum.
The analyte molecules are now in a gaseous state and are bombarded by free electrons generated by a heating filament biased at a negative voltage (-70eV) relative to the source.
The process results in fragmentation of the molecule and generates a positively charged molecule
An EI mass spectrum usually contains the positively charged molecular ion, and many fragment ions, making EI useful for structural characterization of small molecules.
Figure 2 below provides a basic schematic illustration of how EI works.
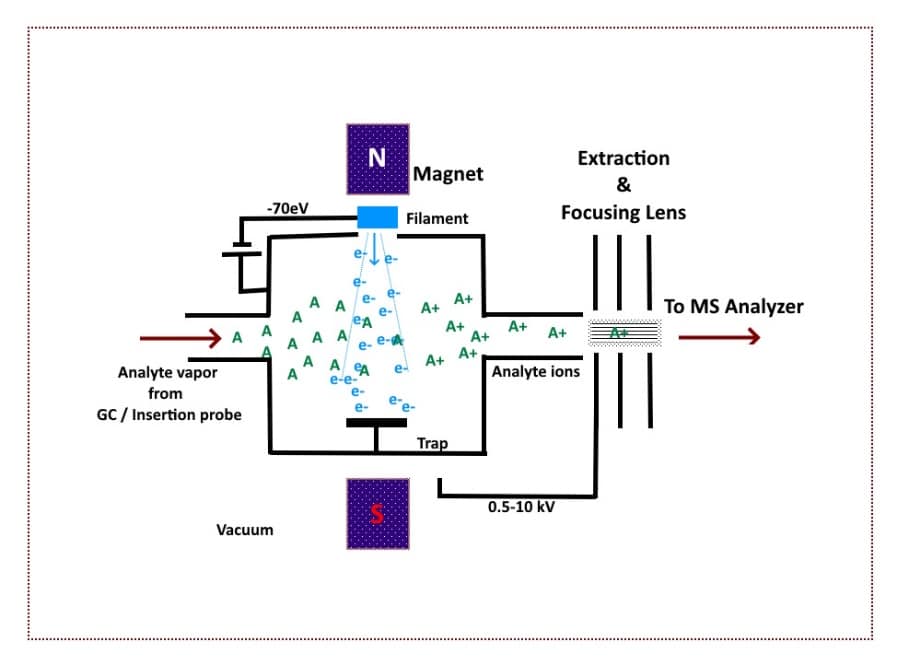
Figure 2. A Schematic of electron impact ionization. (Image credit: Vishal Srivastava.)
Chemical Ionization (CI): Soft Ionization
Chemical Ionization (CI) uses a reagent gas, such as methane, isobutene, or ammonia, to assist in the ionization of the analyte molecule. The ion source device is more powerful than the device used for EI.
This technique is preferred when no molecular ions are observed after using EI or when molecular weight determination of the analyte is needed. [4]
It is more suitable for relatively volatile samples and is particularly effective for the ionization of polar molecules.
The gas is introduced first, and free electrons bombard the reagent gas molecules to create reagent-specific ions (known as reagent plasma; RH+).
Upon sample introduction, sample molecules undergo ion-molecule reactions with the reagent plasma, producing sample ions. [5]
Figure 3 below provides a basic schematic illustration of how chemical ionization works.
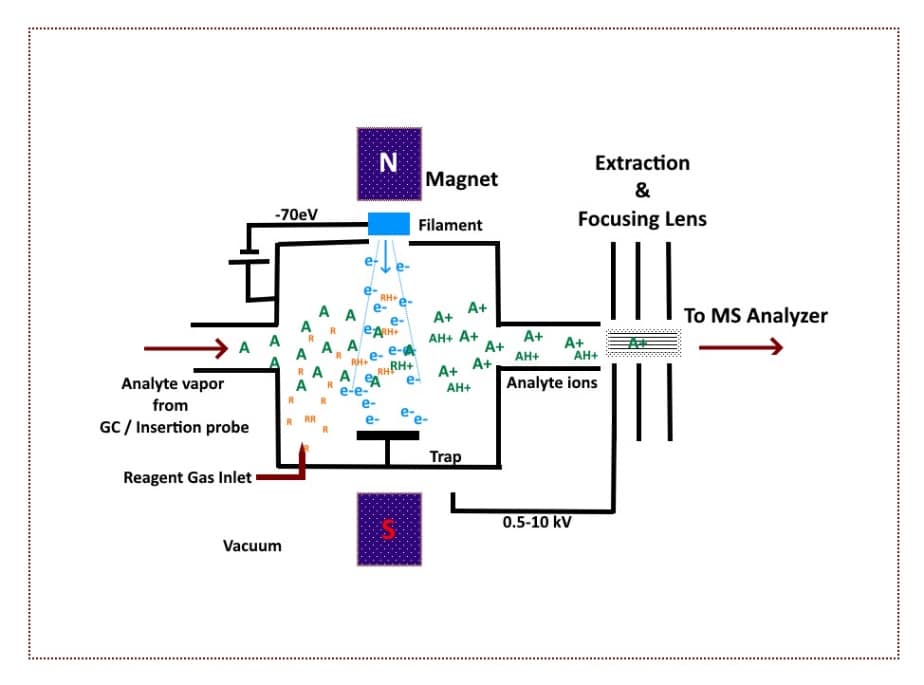
Figure 3. A Schematic of the chemical ionization (CI) process in mass spectroscopy. (Image credit: Vishal Srivastava.)
Fast Atom Bombardment (FAB)
This technique is similar to EI. However, it uses an accelerated beam of atoms (usually Xenon or Argon) to ionize the sample.
The technique was very popular in the 80s to early 90s for ionizing non-volatile compounds.
In fast atom bombardment, the sample is typically mixed with a matrix such as glycerol and 3-nitro-benzoic acid.
The ions generated through the FAB method, protons, sodium (Na+), potassium (K+), and ammonium (NH4+) often form adducts with the analyte. (Adduct simply means bonded together without gaining or losing any atoms or electrons; see the FAQ section below for further information on whether they are a problem and how you can account for them.)
Another variation of the FAB method involves replacing the atom beam with cesium ions. This is referred to as Secondary Ion Mass spectroscopy (SIMS).
Figure 4 below gives a basic schematic illustration of how FAB ionization works.
Figure 4. A Schematic representation of Fast atom bombardment (FAB) ionization. (Image credit: Vishal Srivastava.)
Atmospheric Pressure Chemical Ionization (APCI)
APCI (Atmospheric Pressure Chemical Ionization) is a modern ionization method frequently preferred over the traditional methods discussed above because of its efficiency, ease of ionization, and lesser adduct formation.
The sample is injected into the ionization chamber as a spray. The spray is generated by the flow of liquid combined with a heated inert gas, known as the nebulization gas, which volatilizes the sample. [5]
A needle connected to a high-voltage source is positioned in the ionization chamber. When high voltage is applied at atmospheric pressure it creates a corona discharge—an electrical discharge generated due to the ionization of gas and solvent molecules nearby the conductor needle.
Ions are formed through proton transfer from water clusters present in the sample.
The APCI technique offers ease of use, and the chances of contamination are greatly reduced when performing simultaneous analyses in comparison to other ionization methods.
Figure 5 below gives a basic schematic illustration of how ACPI works.
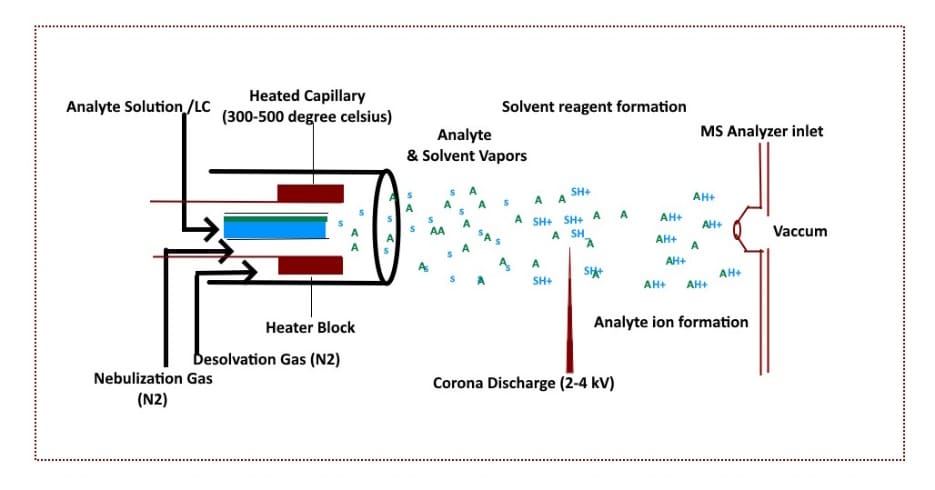
Figure 5. Schematic of the atmospheric pressure chemical ionization (ACPI) method. (Image credit: Vishal Srivastava.)
Electrospray Ionization (ESI)
Similar to APCI, the ESI method applies the sample as a fine spray of liquid at atmospheric pressure. However, instead of using a corona discharge needle, high voltage is applied directly to the flow of the liquid.
The spray is directed into an opening in the vacuum system of the mass spectrometer, where droplets are de-solvated (removal of the solvent) through a combination of heat, vacuum, and acceleration from the applied high voltage.
Eventually, the ions are ejected from the droplets and accelerated into the mass analyzer.
ESI can produce single or multiply charged ions dependent on the size, chemical composition, and higher-order structure of the analyte molecule, as well as the solvent composition, the presence of co-solutes, and the instrument parameters. [5]
ESI is effective for ionizing and analyzing large macromolecules, and is a good choice for analyzing biochemical compounds, including peptides and proteins, lipids, oligosaccharides, oligonucleotides, and bio-organic compounds.
Figure 6 below provides a basic schematic illustration of how ESI works.
Figure 6. Schematic of electrospray ionization in mass spectrometry. (Image credit: Vishal Srivastava.)
Matrix-Assisted Laser Desorption Ionization (MALDI)
Matrix-assisted laser desorption/ionization (MALDI) as a soft ionization method allows effective ionization of high molecular weight compounds with relative masses up to 300kDa, like organic macromolecules such as proteins.
The method requires the co-crystallization of the sample analyte with a large excess of a matrix such as sinapic acid, α-cyano-4-hydroxycinnamic acid), or 2,5-dihydroxybenzoic acid to avoid sample damage by laser irradiation while ensuring efficient ionization of the analyte.
This means that, unlike ESI, the sample is ionized from a solid matrix. MALDI usually also produces fewer ions with multiple charges (doubly protonated or more) which makes it easier to correctly determine the mass of the ions.
Upon laser irradiation of the matrix-analyte preparation, the matrix desorbs and carries the analyte into the gaseous phase. The matrix also serves as a proton donor and acceptor, resulting in the ionization of the analyte sample. [5,6]
If you’re unsure whether to pick MALDI or ESI as your ionization method, check this comparison by Nadler, Waidelich, and Kerner et al. that examines the effect of these ionization methods on peptide identification. [7]
Figure 7 below provides a basic illustration of how MALDI works.
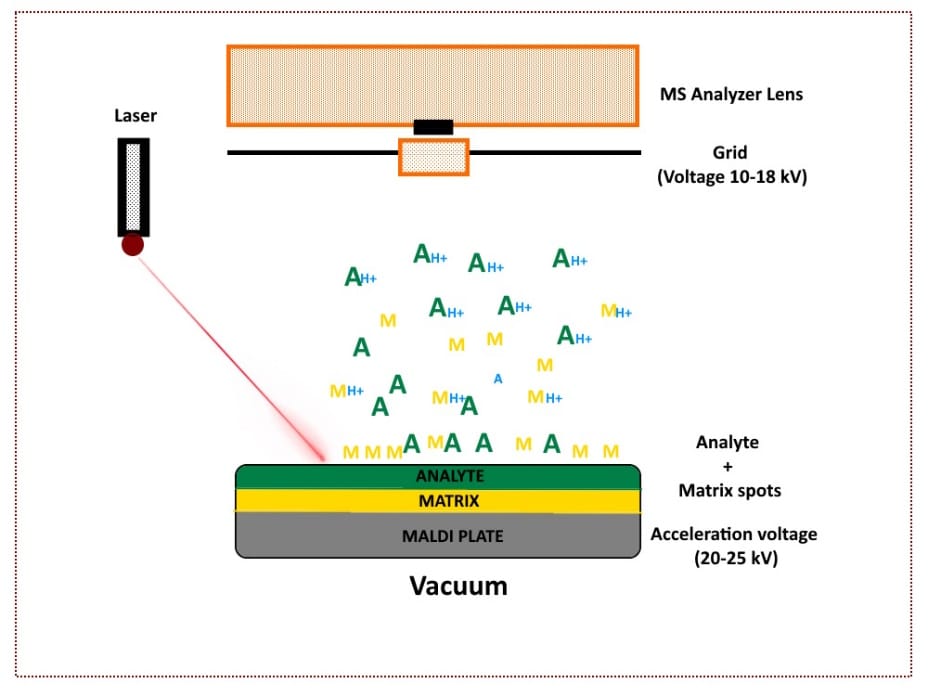
Figure 7. Schematic diagram of MALDI in mass spectrometry. (Image credit: Vishal Srivastava.)
FAQs
Q. Are adducts a problem for mass spectrometry?
A. Adducts in mass spectrometry can indeed affect data interpretation, especially if the adducts introduce unexpected masses or interfere with the isolation of specific molecular ions.
But adduct formation isn’t always problematic—it depends on the analysis goals and the ionization method in use.
For some ionization methods, adduct formation leveraged (as discussed above) to enhance signal detection and improve ionization efficiency. When they are an issue, various approaches, such as using solvents with fewer adduct-prone ions or adjusting ionization conditions, can help mitigate their impact.
When an adduct is of a known mass—such as a molecular ion plus sodium—it can often be accounted for during analysis by calculating expected shifts in mass. This approach helps analysts differentiate between actual molecular ions and adducts, allowing them to isolate the desired molecule’s mass accurately.
When adducts are unwanted, adjustments to the ionization environment or using solvents that reduce adduct formation can help minimize their presence.
Try altering the mobile phase in liquid chromatography or optimizing ionization conditions in the mass spectrometer to help control adduct formation.
Q. How do you choose the best ionization method for a specific type of biomolecule?
A. Choosing the right ionization method for a specific biomolecule requires careful consideration of the molecule’s size, volatility, and stability. EI works well for small, stable molecules but is generally too harsh for biomolecules like proteins.
On the other hand, ESI and MALDI are ideal for larger, less volatile biomolecules, as they produce ions with minimal fragmentation.
Considering the molecule’s sensitivity to fragmentation and the desired level of detail in molecular identification can guide the selection of an appropriate ionization technique.
Q. How does the choice of ionization method impact the overall sensitivity and resolution of the mass spectrometry analysis?
A. The choice of ionization method significantly impacts both the sensitivity and resolution of mass spectrometry analysis.
Different ionization techniques can produce varying amounts of ionization efficiency for different analytes, which affects the overall sensitivity.
ESI is particularly good for large biomolecules like proteins and peptides, allowing for the detection of low-abundance species, thus enhancing sensitivity.
Methods like EI may be less sensitive for larger molecules due to their hard ionization process that often leads to extensive fragmentation.
The resolution of the mass spectrometry analysis can be influenced by the type of ionization method used.
Soft ionization techniques like MALDI generally provide better resolution for larger, more complex biomolecules by minimizing fragmentation, which helps maintain the integrity of the original ion.
Conversely, hard ionization methods may complicate spectra interpretation due to fragmentation patterns, potentially hindering resolution
Molecular Ionization for Mass Spec. It’s a Wrap
That was a brief explanation of the different types of ionization methods in mass spec.
EI is great for small stable molecules but can produce too much fragmentation of larger molecules such as proteins to make it a good choice.
For biomolecules, ESI or MALDI is usually a safe bet.
With this information, you should now be able to decide on a mass-spec method to suit your molecule while meeting the sensitivity and resolution requirements of your experiments.
References
- Baker M (2010). Mass spectrometry for biologists. Nat Methods. 7:157–61
- Ashcroft AE. An Introduction to Mass Spectrometry. URL: https://silo.tips/download/an-introduction-to-mass-spectrometry-3 Accessed 30th of October 2024.
- Greg McMahon. Types of Ion Detector for Mass Spectrometry. Technology Networks, Analysis and Separations
- Urban PL (2016). Quantitative mass spectrometry: an overview. Philos Trans A Math Phys Eng Sci. 374:2079
- Mass spectrometry ionization methods. Emory University. URL: https://chemistry.emory.edu/msc/tutorial/mass-spectrometry-ionization.html Accessed 30th of October 2024.
- Ionization modes. Mass Spectrometry & Proteomics Facility, University of Notre Dame. URL: https://massspec.nd.edu/instruments-and-analyses/ionization-modes/ Accessed 30th of October 2024
- Nadler, Waidelich, Kerner, et al. (2017). MALDI versus ESI: The Impact of the Ion Source on Peptide Identification. J Proteome Res. 16(3):1207–15