Next-generation sequencing (NGS) is a collection of technologies that enables you to identify genetic variations and mutations via RNA and DNA sequencing. It can give you a great range of insights, from single nucleotide variants to comprehensive cancer genome profiles for cancer research, helping you understand tumor progression and heterogeneity.
However, some NGS approaches can be inaccessible due to high costs, limited expertise, and insufficient data management and storage resources. Choosing the wrong NGS approach can lead to wasted resources, inaccurate data, and missed opportunities to uncover critical insights, ultimately delaying your research and any actions on its outcomes.
This article introduces the different NGS options and their applications and explores some of the commercial resources available for streamlining NGS, from sample preparation to data analysis.
What is Next-generation Sequencing?
Next-Generation Sequencing (NGS) is a high-throughput sequencing technology that allows researchers to determine the order of nucleotides (A, T, C, and G) in DNA or RNA molecules. It is a powerful tool used to study genomes, transcriptomes, and epigenomes, providing insights into genetic variations and biological functions.
In cancer research, NGS plays a critical role by:
- Identifying actionable mutations for targeted therapies
- Uncovering tumor heterogeneity and resistance mechanisms
- Supporting biomarker discovery
Benefits of Next-generation Sequencing in Cancer Research
By unraveling genomic profiles of different types of cancer, NGS enables the identification of important genetic variations.
NGS-driven precision cancer research can contribute to the discovery of new cancer targets and biomarkers and provide the information needed to navigate the complex landscape of cancer. The main benefits of NGS include:
- High throughput: NGS can analyze multiple samples in a single run, saving time and resources compared to traditional sequencing methods.
- Comprehensive analysis: NGS allows for the simultaneous detection of a wide range of genetic alterations, providing a complete picture of a tumor’s genetic profile.
- High analytical sensitivity: It can identify rare mutations and low-frequency variants, critical for understanding tumor heterogeneity and resistance mechanisms.
- Cost-effectiveness: Reduces the cost per base of sequencing compared to earlier technologies.
- Supports tumor-agnostic approaches: By focusing on molecular alterations rather than the tissue of origin, NGS facilitates the development of cancer treatments driven by similar genetic changes, regardless of tumor type.
- Advances precision medicine: NGS enables the identification of actionable mutations and biomarkers, allowing researchers to develop potential therapies that could target the specific genetic drivers of a cancer specimen.
The Role of Next-generation Sequencing in Precision Medicine
In cancer research, precision medicine aims to identify the genetic mutations driving a tumor’s growth and to use this information to identify potential improvements to current therapies
NGS is pivotal in enabling precision medicine by providing a comprehensive and high-throughput method to analyze cancer’s genetic and molecular landscape and therefore supporting the discovery of actionable biomarkers.
Challenges of Using Next-generation Sequencing in Cancer Research
Despite the promise that NGS holds for advancing research in cancer research, several factors have slowed progress in this field:
- Cost of equipment and resources: The high upfront cost of sequencing instruments, reagents, and consumables can be prohibitive. [1] Additionally, NGS requires expensive computational power and data storage capacity.
- Limited access to the latest cancer research: NGS tools and methodologies can be inaccessible, either due to geographic disparities, inadequate funding, or lack of established collaborations with global cancer research initiatives. [1]
- Requires bioinformatics expertise for data analysis: The complex datasets generated by NGS require advanced bioinformatics skills to process, interpret, and derive meaningful insights.
- Challenges with sample quality and preparation: Low-quality or degraded samples can complicate library preparation and lead to unreliable results, requiring optimized protocols or specialized kits to ensure high-quality data.
- Time-intensive workflows: While NGS has high throughput, the end-to-end process can be time-consuming, from sample preparation and sequencing to data analysis.
Next-generation Sequencing Tools for Cancer Research: What Are Your Options?
Single Gene Testing
Single gene testing (also referred to as whole gene testing) is a technique that analyzes one specific gene or a small part of a gene to identify mutations or alterations that may be associated with a particular disease, such as cancer. For example, single-gene testing is used to identify mutations in the BRAC1/2 gene, a common driver of oncogenesis in breast cancer. [2,3]
Examples of single gene testing techniques include:
- Fluorescence and chromogenic in situ hybridization (FISH)
- Polymerase chain reaction (PCR)
- Microsatellite instability (MSI) tests
Single-gene testing is a well-established technique. It offers the advantage of simple, streamlined analysis and is cost-effective.
Be aware that single-gene testing approaches can fail to capture the complete picture of mutations driving tumorgenesis since some cancers result from an accumulation of mutations across multiple genes. Consider combining single gene testing with more advanced techniques.
Small to Large Panel Testing
Small and large panel testing are robust NGS approaches that enable you to analyze multiple genes simultaneously, offering a scalable solution for identifying cancer-associated mutations.
Small gene panels (< 50 genes) target a select set of genes previously identified as having relevant mutations in specific cancer types. [1] Small panel testing will offer more information per sample than single gene testing while remaining cost-effective with streamlined data analysis and interpretation.
Large panel testing (> 50 genes) targets various cancer-associated genes. [1] This genetic profiling approach is beneficial if your sample is a liquid biopsy or tumor tissue. Increasing the scope of sequencing via large gene panels further reduces the risk of missing key mutations and captures a more comprehensive view of the tumor’s genetic landscape.
Advanced Next-generation Sequencing Techniques
Advanced NGS methods will provide you with an in-depth and broad analysis of genetic and transcriptomic data, giving you insights into the genomic signature in cancer. Examples of advanced NGS techniques include:
- Comprehensive genomic profiling (CGP)
- Whole exome sequencing (WES)
- Whole genome sequencing (WGS)
- RNA sequencing (RNA-seq)
- Whole genome and whole transcriptome sequencing (WGTS)
These advanced approaches are summarized in Table 1 below.
Table 1. Summary of the different advanced NGS techniques.
Approach | Description | Benefits | Limitations |
Comprehensive genomic profiling (CGP) | Tumor agnostic approach which gives a comprehensive overview of the cancer biomarker landscape.
| The multiplexed approach can save time and money and reduce the need for sequential biomarker testing.
| Access to this method can be challenging. |
Whole exome sequencing (WES) | Targets the protein-coding regions of a genome (called the exome), which have been shown to contain up to 85% of disease-associated variants. [4] | Cost-effective. Targets the portion of genes known to contain a majority of mutations. | Can miss relevant variants located outside of the exome.
|
Whole genome sequencing (WGS) | Generates sequence data for the entire genome. | Generates sequencing data for the entire genome. Will allow you to detect mutations in the non-coding regions of the gene. | More expensive than WES.
|
RNA sequencing (RNA-seq) | Analyses the transcriptome. Provides you with information on splice variants, fusion transcripts, and other transcriptional biomarkers associated with cancer. | This technique captures all RNA types, including coding and non-coding RNAs.
| RNA-seq results are impacted by sample quality and rely on high-quality sample preparations.
|
Whole genome and whole transcriptome sequencing (WGTS) | A multidisciplinary approach that combines WGS with RNA-seq. | Gives you a comprehensive overview of the genome and transcriptome. | WGTS is expensive, data-intensive, and requires you to have informatics expertise.
|
Choosing the Right Next-generation Sequencing Approach for Your Research
In general, with NGS sequencing, there is a trade-off between the information gathered and the cost and accessibility of the approach (Figure 1).

When deciding which NGS approach to use for identifying an actionable mutation, consider the following:
- What is your research objective? A smaller, more targeted sequencing panel will be appropriate if your mutation of interest is well-defined. Choose methods such as WES, WGS, or RNA-seq if researching novel mutations.
- What is your budget? Larger data sets will cost more than targeted panels. Don’t forget to consider any costs associated with handling large quantities of data.
- Sample quality: Is your sample source high quality enough for your method of choice?
- Depth of sequencing: You should choose a high-depth approach (such as a targeted panel) for detecting rare variants, characterizing heterogeneous tumor populations, and measuring low-level variants. The low-depth analysis is applicable for broad genomic analysis.
- Time available: A targeted sequencing panel will provide you with an answer much quicker than a more comprehensive sequencing approach.
- Resource availability: When choosing your NGS approach, ensure you can access the necessary tools and resources for the data analysis. The comprehensive sequencing techniques (WES, WGS, RNA-seq, and WGTS) will require bioinformatic expertise and capacity to store large data sets.
Consider a Multi-faceted Approach for Comprehensive Analysis
While some methods, like CGP assays, can stand alone to characterize cancer samples, others are most effective when applied in tandem to support your research.
By combining techniques like WGS, RNA-seq, and WES, you can correlate DNA mutations with RNA expression, identify novel biomarkers, and uncover regulatory mechanisms driving cancer.
Tumor-informed minimal residual disease (MRD) research is an example of an integrated NGS approach. This involves sequencing tumor samples via WES. The results of the WES sequencing are then used to design a custom gene panel to sequence at a more profound level.
Figure 2 below illustrates the information vs. accessibility trade-off between NGS approaches in cancer research and shows some of their key parameters.
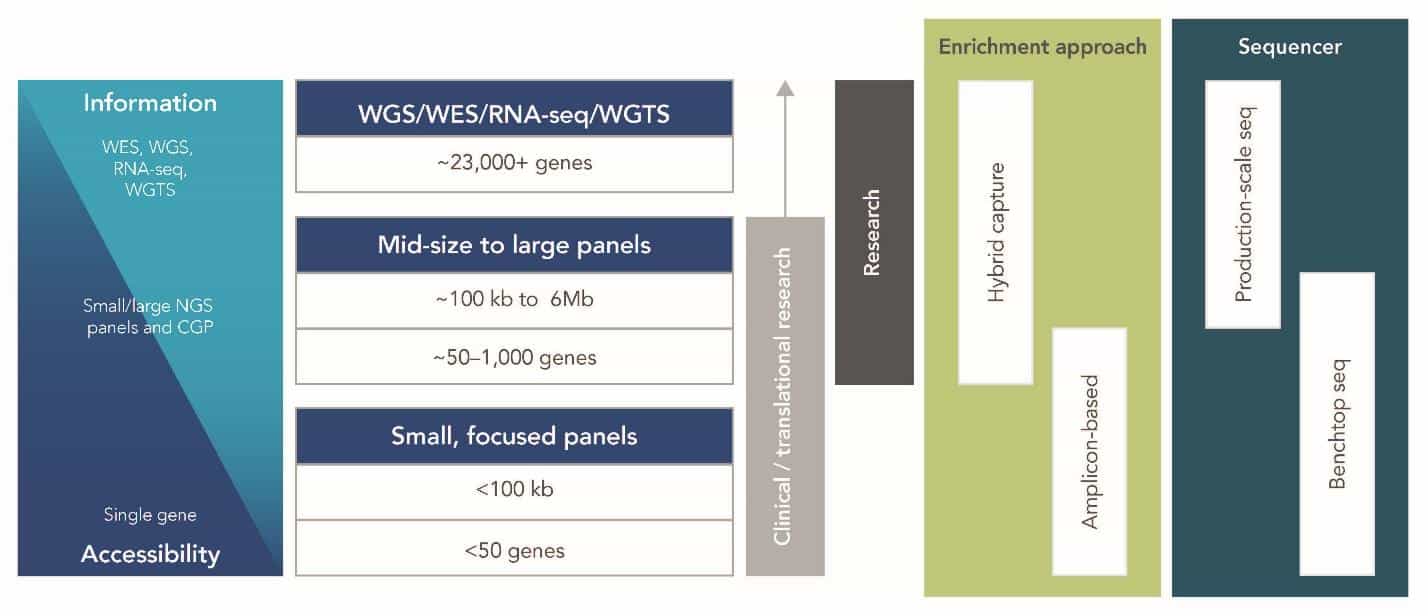
Building Optimal Next-generation Sequencing Approaches Using IDT Workflows
IDT offers a range of tools designed for precise and reliable NGS to assist cancer research at every stage.
Features of their integrated workflow solutions include custom enrichment panels and design services to streamline the detection of tumor-specific mutations in challenging sample types, such as cell-free DNA (cfDNA) and Formalin-Fixed Paraffin-Embedded (FFPE) tissues.
In addition, IDT offers integrated analysis software, simplifying data interpretation and allowing you to process complex datasets quickly and efficiently.
IDT will provide end-to-end support, from optimizing your sample preparation to automating your data analysis so you can focus on your research instead of troubleshooting. Click here to get started.
Summary of Solutions for Next-generation Sequencing
Challenges of NGS include high costs, complex workflows, and the need for specialized expertise to manage and analyze large datasets.
Selecting the most appropriate NGS approach and leveraging streamlined, customizable workflows will help you overcome these and gain actionable insights into cancer biology.
IDT provides comprehensive solutions, including tailored enrichment panels, user-friendly tools, and integrated data analysis, making advanced NGS approaches more accessible, efficient, and reliable for cancer research.
Sign up to IDT Insider to be among the first to learn about new product announcements, register for upcoming webinars, and even read compelling employee spotlights.
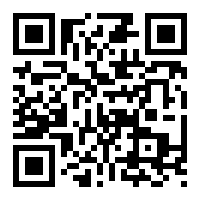
References
- Bayle A, Bonastre J, Chaltiel D, et al. (2023). ESMO study on the availability and accessibility of biomolecular technologies in oncology in Europe. Ann Oncol. 34(10):934–45
- Miki Y, Swensen J, Shattuck-Eidens D, et al. (1994). A strong candidate for the breast and ovarian cancer susceptibility gene BRCA1. Science. 266(5182):66–71
- Wooster R, Bignell G, Lancaster J, et al. (1995). Identification of the breast cancer susceptibility gene BRCA2. Nature. 378(6559):789–92
- Ng SB, Turner EH, Robertson PD, et al. (2009). Targeted capture and massively parallel sequencing of 12 human exomes. Nature. 461(7261):272–76
- Nakagawa H, Fujita M. (2018). Whole genome sequencing analysis for cancer genomics and precision medicine. Cancer Sci. 109(3):513–22