DNA cloning is where biotechnical innovation begins. From synthetically produced enzymes to new crop varieties, microbial strains, biodegradable materials, biofuels, and so much more—it all begins with DNA cloning techniques.
Planning a DNA cloning experiment can be overwhelming without understanding the many strategies available to optimize workflows. This article compares different approaches to molecular cloning and synthetic DNA assembly methods so that you can make informed choices about your cloning experiments and execute them well.
What is Meant by DNA Cloning?
DNA cloning (also called molecular cloning) is an umbrella term for laboratory techniques used to create a population of organisms that all carry the same DNA sequence (clones). [1]
At its core, DNA cloning involves three main aspects:
- Inserting a DNA sequence of interest into a vector;
- Transforming the vector into the host system;
- Utilizing the host’s expression systems to produce the target protein.
The general workflow includes the following steps outlined in Figure 1.

Figure 1. Basic workflow for DNA cloning techniques. [2]
Choosing Your Vector
Vectors are circular DNA molecules that carry your DNA sequence of interest. There are two main functional types:
- Cloning vectors: These vectors typically contain essential elements such as an origin of replication, multiple cloning sites for DNA-insertion, and an antibiotic resistance gene to select for cells carrying the vector.
- Expression vector: These vectors contain host-specific regulatory elements such as promoter and terminator sequences that drive protein expression of the target protein.
Bacterial plasmids are the most common vectors. Other types include viral vectors (bacteriophages), bacterial artificial chromosomes (BACs), yeast artificial chromosomes (YACs), and mammalian cell-specific vectors. [3]
Designing Your Sequence of Interest
Your DNA sequence of interest can be artificially synthesized or isolated using polymerase chain reaction (PCR).
You can use a single DNA fragment or assemble multiple fragments for your cloning experiment. If you choose to assemble multiple fragments, it is more convenient and faster to do this synthetically. This approach eliminates the need to amplify or piece together shorter regions from several templates, streamlining the process significantly.
In addition, you can also tailor your sequence to have features like restriction enzyme sites, phosphate groups, or complementary sequence overlaps to facilitate the assembly process. These design considerations will change according to how you intend to create your DNA-vector construct (also known as a recombinant vector).
Creating Your DNA-vector Construct
Creating a DNA-vector construct, or recombinant vector, involves combining your DNA sequence of interest (your insert) with your chosen vector. A common approach is the ‘cut and paste’ method, which uses restriction enzymes and DNA ligase to join the DNA and vector.
Newer techniques, such as seamless cloning and Golden Gate assembly, bypass the need for restriction enzymes but require advanced design of the DNA fragments. Understanding the strengths and limitations of each method is essential for selecting the best approach for your research.
In the following sections, we will describe and compare these methods to help you make the most informed decision.
Blunt-end cloning
Blunt-end cloning is a quick and straightforward method that uses restriction enzymes to create blunt ends in the DNA strands, which have no overhanging bases. However, the method is non-directional, meaning the DNA can be inserted in random orientations. This makes it less suitable for assembling multiple fragments, as one or more of the fragments might be inserted in the wrong direction.
To improve the efficiency of blunt-end cloning, it is best to prepare DNA with 5’-phosphate ends, as these groups are essential for ligation with the vector. Additionally, removing 5’-phosphates from the vector prevents it from self-ligating, ensuring that it only joins with the insert.
TA Cloning
TA cloning is another quick and easy method. Instead of restriction enzymes, Taq polymerase adds A and T nucleotide overhangs, creating sticky ends in the DNA strands and vector. This method is non-directional, making it best suited for single-fragment constructs.
Synthetic DNA fragments can be ordered as-is, and A-overhangs can be added later using Taq polymerase. For PCR-amplified fragments, A-overhangs can be included directly in your primers.
Restriction Enzyme Cloning
Similarly to TA cloning, restriction enzyme cloning creates short nucleotide overhangs in the DNA strands and the vector. While this method offers directional cloning, it is not ideal for assembling multiple fragments because it relies on specific enzyme recognition sites to cut and join DNA.
To enhance this method, design DNA fragments with recognition sites for restriction enzymes and add 4–5 extra bases to improve enzyme binding.
Golden Gate
The Golden Gate method allows for the assembly of constructs containing multiple DNA fragments without leaving “scars” (residual bases that could impact protein expression). This method is particularly well-suited for assembling fragments with internal structural complexities.
However, enzyme recognition sites must be carefully placed to avoid undesired cuts, and similar overhang sequences can reduce efficiency by causing unintended ligation.
Seamless Cloning
As with Golden Gate cloning, the seamless method assembles multiple DNA fragments without leaving scars. However, it is sensitive to sequence complexities, such as GC-rich regions, repetitive sequences, or secondary structures, which can hinder the assembly process.
Gateway Cloning
The Gateway cloning method (Thermo Fisher Scientific) facilitates the transfer of DNA fragments into destination vectors using homologous (att) sites. This process is quick and efficient, and it does not require ligase.
A summary of each method can be seen in Figure 2, and the pros and cons of each method are described in Table 1.
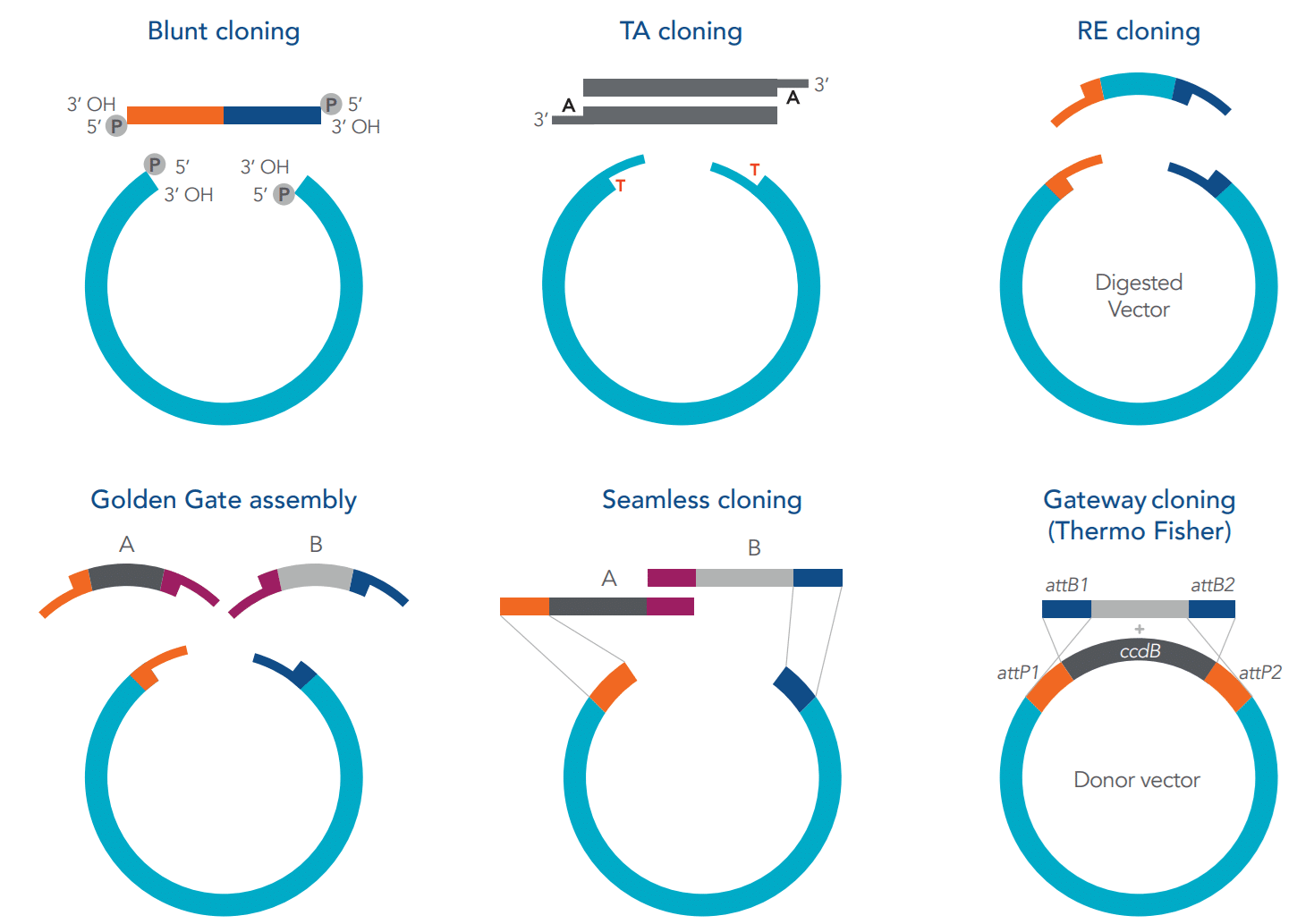
Figure 2. Summary of each cloning method. [2]
Table 1. Summary of cloning methods. [2]
Assembly Method | Pros | Cons |
Blunt-end cloning TA cloning | Fast and easy No extensive preparation or design steps | Non-directional cloning Often requires more screening Not suitable for assembling larger constructs |
Type II restriction enzyme cloning (sticky ends) | Directional cloning with high efficiency Unique restriction enzyme cut sites ensure correct ligation Widely available restriction enzymes and buffers | Requires restriction enzyme cut sites in both insert and vector Leaves “scars” or residual bases in the construct |
Type IIS restriction enzyme cloning (Golden Gate assembly) | Scarless cloning as type IIS enzymes cleave outside the recognition site Sticky ends for highly efficient cloning and ligation Good method for assembling multiple fragments | Requires restriction enzyme cut sites in both insert and vector May result in non-specific ligation if overhangs of fragments are similar (only differ by a base or two) |
Seamless cloning | Fast cloning of single or multiple fragments in a single assembly reaction Does not require restriction sites Highly efficient | Requires overlaps of 15—40 base pairs between sequences of fragments, inserts, and vectors |
Gateway cloning | Rapid exchange of inserts between vectors Large selection of Gateway-compatible plasmids available | Requires att sites at flanking ends of fragments, so leaves “scars” Cannot be used to assemble multiple fragments |
Designing and Assembling Synthetic DNA Fragments
Why Assemble Multiple DNA Fragments?
Assembling multiple fragments allows you to achieve sequence lengths of up to 3 kb. These longer sequences can accommodate a variety of elements, including protein-coding regions, regulatory elements, biological parts, antibody fragments, genomic regions, and more.
The Challenges of Assembling DNA Fragments
Large constructs often come with sequence complexities that can interfere with the synthesis, assembly, or end use of DNA fragments. Common complexities and their management strategies are listed below and expanded upon in Table 2.
- Extremes in GC content: Manage with a stabilizing sequence.
- Repeat regions: Manage by creating sub-fragments.
- Secondary structures: Manage with codon optimization.
Table 2. Summary of strategies to reduce complexities of DNA sequences. [2]
Adding stabilizing sequences | Splitting into sub-fragments | Codon optimization |
Add extra bases to fragment terminal ends | Create sub-fragments of the original sequence | Modify DNA sequences while preserving amino acid sequence |
Most effective when complexities are at terminal ends | Most effective when internal complexities are spaced apparat and can be separated | Most effective for complexities spread across the entirety of the DNA sequence |
Stabilizing bases may need to be removed | Sub-fragments need to be reassembled | The optimized DNA sequence will differ from the original sequence |
Consider Using Online Tools to Help Design Cloning Experiments
DNA sequence manipulation software greatly simplifies the design of cloning experiments. These tools can annotate sequences, generate vector maps, analyze GC content, identify structural motifs, calculate melting temperatures, locate restriction enzyme sites, and visualize cloned constructs. This helps ensure you don’t purchase a sequence that won’t be viable.
IDT’s SciToolsTM offers a complexity screening mode that checks input sequences for the three types of complexities described above. It highlights problematic regions within a DNA sequence and provides suggestions for improvement.
Example: Reduce Complexity by Adding a Stabilizing Sequence
Here is an example of how stabilizing sequences can help reduce complexity scores. The test sequence contained a region with high GC content in the 3’ end of the sequence. The complexity tool identified this issue and flagged the sequence as not being viable. Once the stabilizing sequence (in red) was added, the complexity score was reduced to moderate, making the sequence viable for purchase (Figure 3).
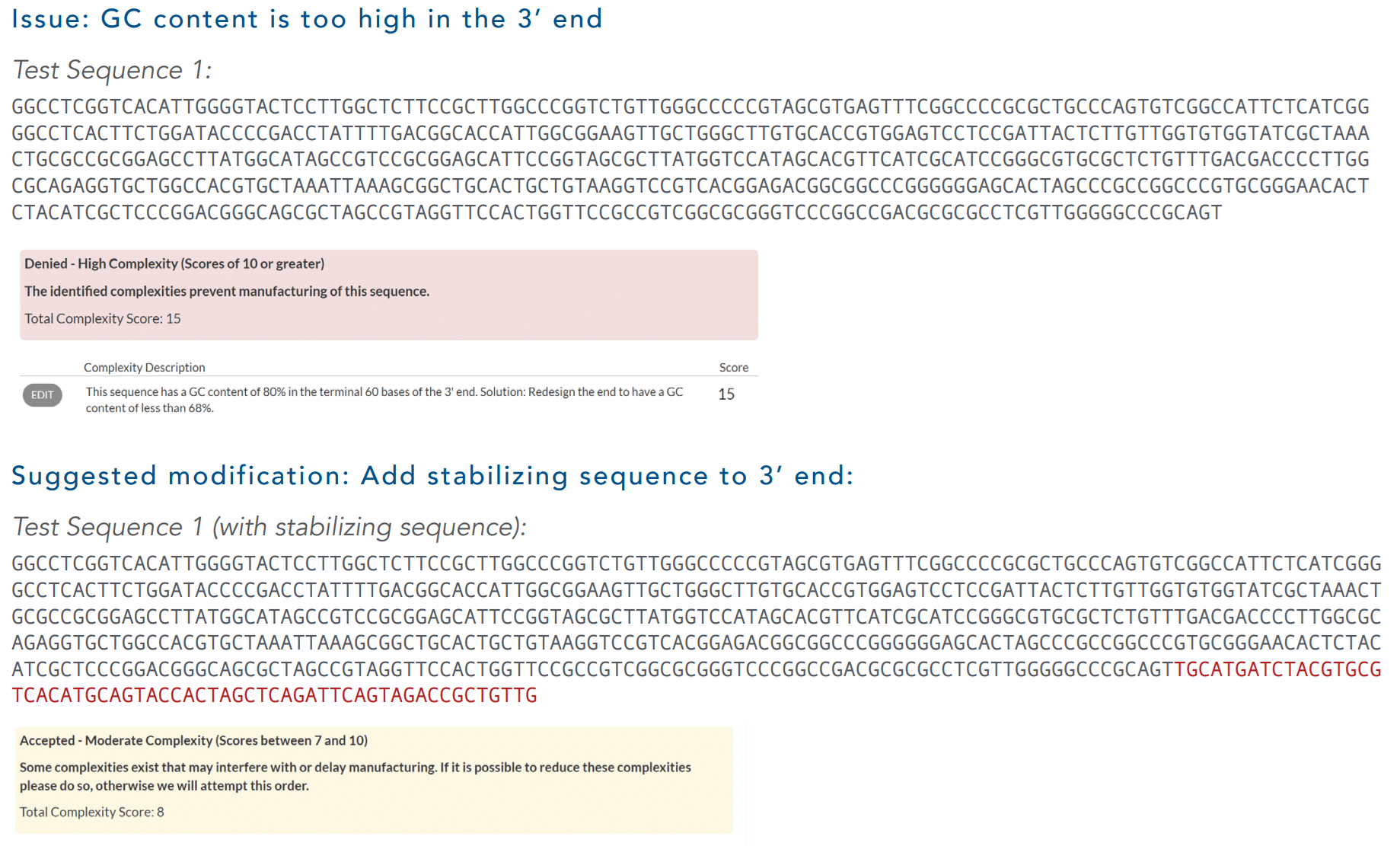
Figure 3. Example of reducing the complexity score of a gBlocks Gene Fragment (IDT) by adding a stabilizing sequence.
DNA Cloning Summarized
DNA cloning is the cornerstone of biotechnology, offering diverse methods to meet various research needs. With so many options available, understanding these methods is essential to overcoming challenges and achieving reliable results.
For more detailed insights into cloning techniques, sequence design, troubleshooting, and IDT’s innovative tools, explore the IDT DNA Cloning Handbook. It’s your go-to resource for making DNA cloning more efficient and effective.
Sign up to IDT Insider to be among the first to learn about new product announcements, register for upcoming webinars, and even read compelling employee spotlights.
References
- Bertero A, Brown S, and Vallier L. (2017). Chapter 2, Methods of cloning. In Basic science methods for clinical researchers (pp. 19-39). Academic Press
- DNA Cloning Handbook. (2022). Integrated DNA Technologies
- Clark DR, Pazdernik NJ, McGehee MR. (2019). Chapter 7, Cloning Genes for Synthetic Biology. In Molecular Biology (pp. 199-239). 3rd Ed