If you think FRET stands for Fluorescence Resonance Energy Transfer, you are wrong. In good company but wrong. FRET actually stands for Förster Resonance Energy Transfer. Find out why and more about how FRET works in this article.
A Short History of FRET
In 2011, it was the centenary of the birth of eminent German physical chemist Theodor Förster. Förster was a very significant figure in his field. His work provided important insights into the nature of excited-state photochemical reactions and the understanding of organic dyes.
His most famous contribution was his work on the electrostatically mediated energy transfer between sensitizer and acceptor molecules. And while it is quite possible that you have never heard Förster’s name before, I bet you HAVE heard of the technique that bears his name: Förster Resonance Energy Transfer or FRET.
One of the reasons for this discrepancy may be because you always thought these initials stood for “Fluorescence Resonance Energy Transfer”, which is OK, but strictly speaking, not entirely correct, as we will see below.
FRET is key to a wide range of natural phenomena, including photosynthesis. However, its application in microscopy and its ability to uncover molecular processes in biological systems at the microscopical level is what I will focus on in this article.
Why Use FRET?
Investigating the interactions between different proteins in a cell is critical for understanding protein function. Certainly, biochemical approaches such as immunoprecipitation and pull-down assays are indispensable for identifying protein-protein interactions.
However, these techniques do not provide spatial resolution or even temporal information in the context of a living cell. On the other hand, in living cells, immunofluorescence can provide spatial resolution, and fluorescent tags, such as GFP, can provide spatial and temporal information in living cells. But these approaches tell us little about protein-protein interactions.
The Challenges with Visualizing Protein-Protein Interactions
It is only logical that to have interaction, there must be colocalization. You could detect colocalization by employing fluorescent antibodies or fluorescent proteins, such as one green protein and a second red protein, and scanning the sample with a confocal microscope, or using deconvolution, or both to identify colocalization or yellow.
But a yellow signal may or may not equal colocalization, depending on your definition of colocalization and specifically what scale you are interested in.
If, for example, you want to know if a certain non-pyramidal neuron expresses both GABA and Parvalbumin, you can stain for both (GABA in red and Parvalbumin in green). If the neuron looks yellow, then you CAN say that these proteins are both in that neuron. But things are not so definitive the closer you look.
When using a diffraction-limited microscope, we must know that we cannot decipher distances that are (at best) less than 200 nm. This means that with these microscopes, any two signals that originate from points closer to each other than 200 nm are considered “colocalized”, not because they are but because of technological limitations.
Second, we must remember that if we are looking for an interaction, this kind of colocalization (vicinity, to be more realistic) is not enough. It would be better to know that the two proteins are actually close enough to interact. Enter FRET.
Principles of FRET
Understanding Fluorescence
Before we dive into the details of how FRET works, you need to have a good understanding of how fluorescence works. In short, fluorescent molecules absorb light within a specific range (their excitation spectrum), causing them to be excited.
The excited phase lasts a short time because the excited molecule wants to return to the low energy state, which is achieved by releasing energy, first through vibrations and then through emitting light (Figure 1).
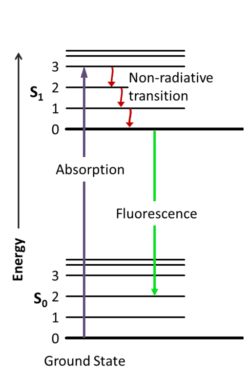
Read the article How Fluorescent Molecules Work for more detail.
How FRET Works
The use of FRET in optical microscopy makes it possible to detect two molecules approaching within the range of a few nanometers. To perform FRET, you need two fluorescent molecules: a donor and an acceptor.
When these molecules are close, and the donor fluorophore is excited, part of its energy is transferred to the acceptor fluorophore. This transfer causes the acceptor fluorophore to fluoresce, even though it has not been directly excited by light (Figure 2).
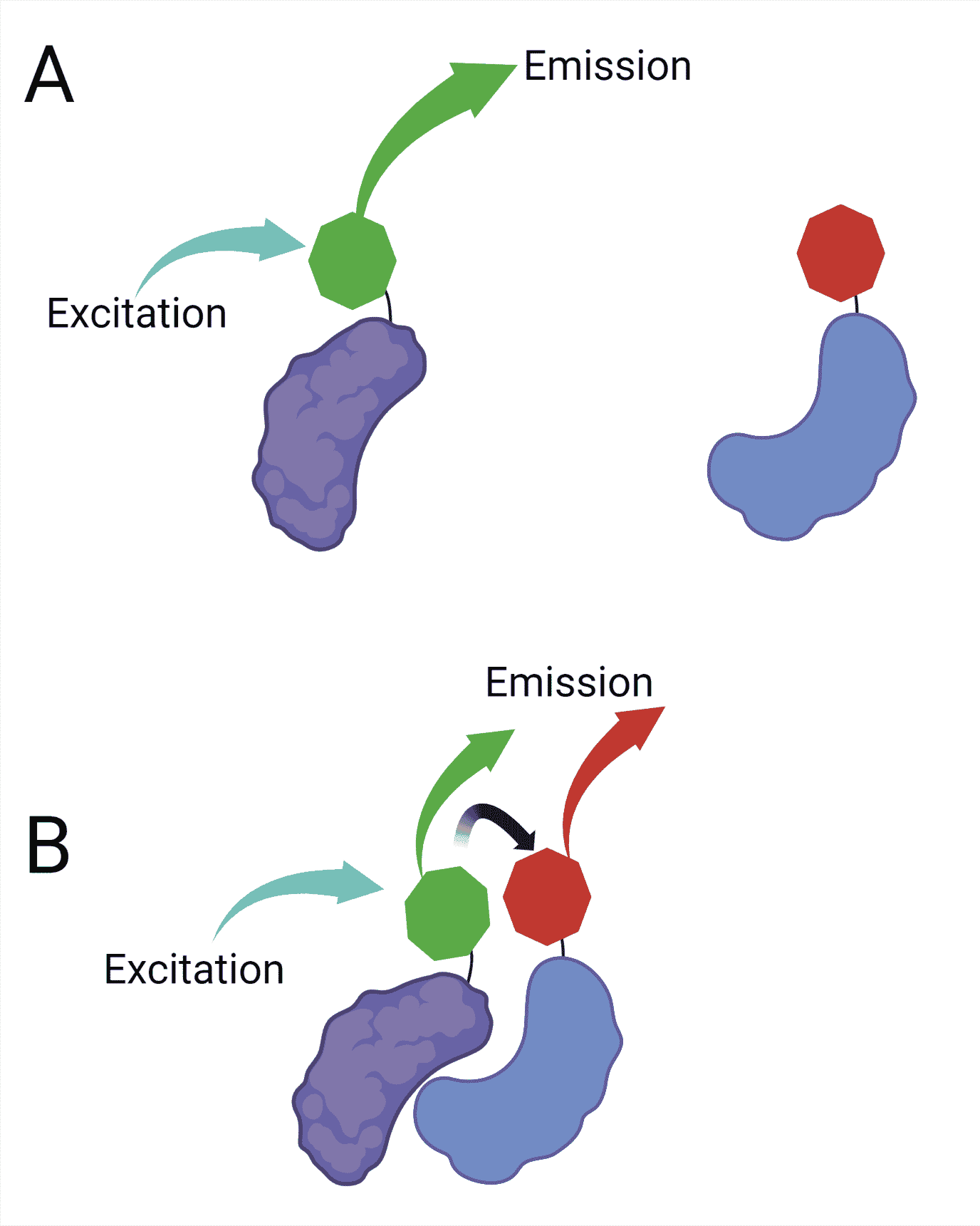
The transfer of energy between the donor and the acceptor happens in a non-radiative fashion through long-range dipole-dipole interactions.
In short, the excited fluorophore acts as an oscillating dipole that can undergo an energy exchange with a second dipole having a similar resonance frequency. This is why calling FRET “Fluorescence Resonance Energy Transfer” is not entirely correct, as it implies that it is through fluorescence that the energy is transferred, which is not the case.
Understanding FRET Efficiency
The range over which this energy transfer can occur is limited to about 10 nm. And as the efficiency of transfer is highly sensitive to the distance between fluorophores, FRET measurements prove very useful for assessing close molecular interactions.
The energy transfer efficiency also depends on the spectral overlap of the two fluorophores. That is the overlap between the donor fluorophore’s emission spectrum and the acceptor fluorophore’s excitation spectrum.
Therefore, you need to take care when choosing your fluorophore pairs for your FRET experiment. For example, CFP and YFP are a very popular FRET pair, and you can easily understand why when you look at their excitation and emission spectra (they overlap well).
Measuring FRET
We cannot directly measure the energy transfer from donor to acceptor. Instead, indirect measurements are used to infer FRET. These include measuring the:
- amount of acceptor excitation.
- level of donor quenching.
- rate of fluorescence decay.
Read the article How to Measure FRET to get details on the different methods for measuring FRET and get practical tips for choosing FRET pairs.
A Summary of How FRET Works
FRET occurs when a donor and acceptor probe are in proximity, and the excited donor transfers energy in a non-radiative manner to the acceptor, exciting the acceptor.
The efficiency of FRET is highly sensitive to distance, making FRET a more accurate way to measure interaction than colocalization.
FRET cannot be measured directly, but there are several indirect ways FRET can be quantified.
We hope this short guide has helped you understand how FRET works. But if you have any questions or thoughts, let us know in the comments section below.
Originally published February 3, 2015. Reviewed and updated October 2022.