Our old friend ethidium bromide (EtBr) has achieved an almost legendary villain status among many molecular biologists, rivaling that of Scar in The Lion King.
The panic regarding its use in the lab has long been a source of irritation for many. Researchers are understandably wary of this potential carcinogen.
However, in recent years, this wariness has been whipped into a witch-hunt by companies advertising “safer” alternatives and disposal methods.
While I don’t for a minute think that we should all throw our gloves away and bathe in the stuff, I think that it’s time for an informed reality check about the utility and dangers of, and myths surrounding, EtBr.
We appreciate that this article goes against the received wisdom that EtBr is extremely dangerous.
At Bitesize Bio, one of our core aims is to engage researchers to think critically about every aspect of their research.
This includes appropriately evaluating received wisdom and “common knowledge”. That’s why we’ve created this article—so you can understand and evaluate the evidence for yourself.
EtBr as a Nucleic Acid Stain: A Serendipitous Invention?
A Short History of EtBr in Animal Medicine
While EtBr has multiple uses, it is perhaps most widely known as a nucleic acid stain [1,2]—but how did it gain such a notorious status in molecular biology?
As you might already know, EtBr (under the trade names Homidium, Ethidium, and Novidium) has been used as a veterinary drug to treat African animal trypanosomiasis in cattle since the 1950s. [3–5]
In areas with a high incidence of EtBr resistance, isometamidium chloride, a derivative of EtBr, is used instead. [6]
The African animal trypanosomiases, caused by infection with Trypanosoma spp., are some of the most significant diseases affecting livestock, equids, and dogs in parts of Sub-Saharan Africa.
In addition, this group of diseases poses a significant obstacle to the socio-economic development of the affected countries. [3,5]
What Makes EtBr Good for Staining Nucleic Acids?
Two physiochemical features of the EtBr structure (Figure 1) have contributed to its success as a celebrity nucleic acid stain; let’s discuss these in more detail.
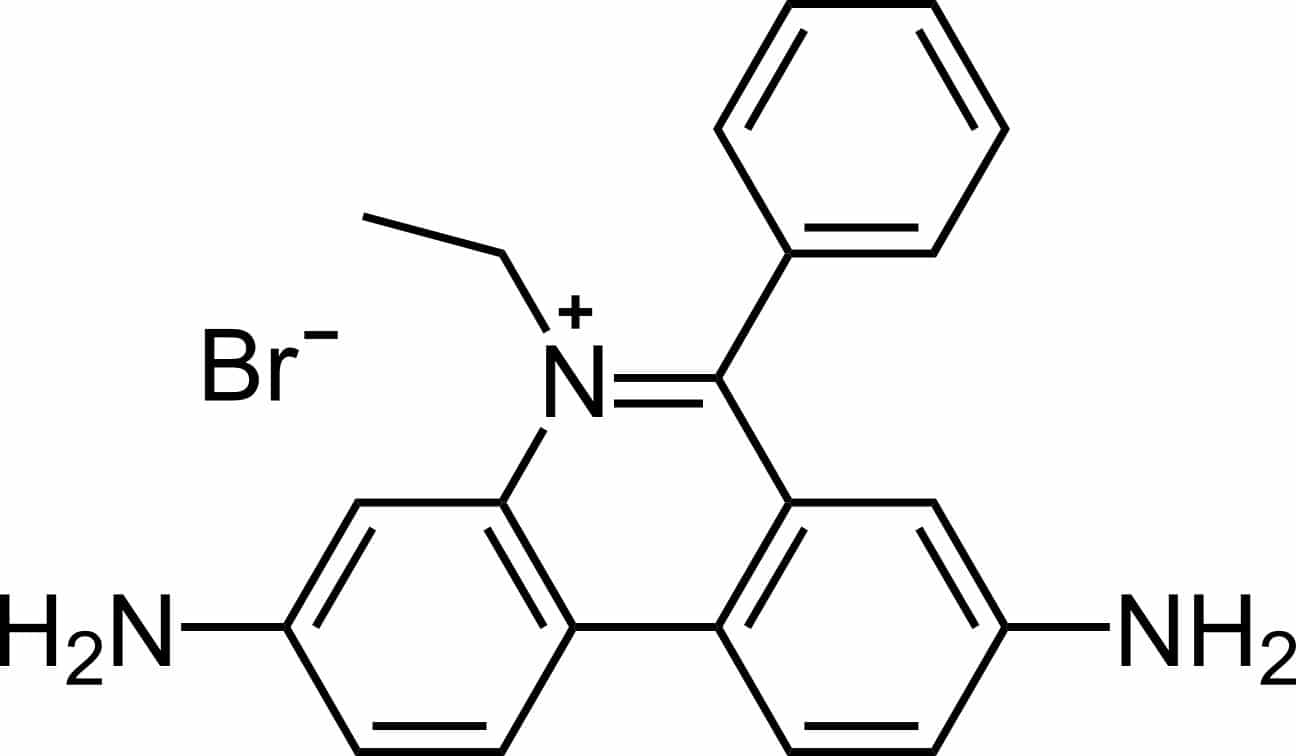
Figure 1. Structure of ethidium bromide. Image credit: Calvero. Public domain, via Wikimedia Commons.
Like many other fluorescent dyes, EtBr is an aromatic compound. It emits a red/orange light at an emission maximum of 560 nm upon excitation with ultraviolet light (maxima ranging from 260 nm to 360 nm). [7,8]
In addition, EtBr is structurally similar to a DNA base pair, allowing it to intercalate into double-stranded DNA (dsDNA). [1,9,10]
EtBr also detects single-stranded RNA (ssRNA) because it folds back on itself, resulting in local base pairing for the EtBr to insert itself into. [1]
On incorporation into dsDNA or ssRNA, EtBr moves from an aqueous to a hydrophobic environment, forcing the EtBr cation to shed any water-associated molecules.
Given that water efficiently quenches fluorescence, its removal allows the intensity of EtBr fluorescence to increase on interaction with a nucleic acid and when excited at the correct wavelength. [1,2]
Some additional applications and working concentrations of EtBr are mentioned in Table 1.
Table 1. Typical uses and working concentrations of EtBr
Purpose | Typical concentration of EtBr |
Veterinary drug for African animal trypanosomiasis (cattle, sheep, goats, pigs) | 1 mg/kg (dose administered to animal) [3,5] 0.075 μg/mL (blood concentration 24 hours post administration) [5] |
In vitro trypanosome cell culture | 10 nM (induces loss of mitochondrial DNA in Trypanosoma brucei) [11,12] |
Agarose gel electrophoresis | 0.5 μg/mL [7,13] |
Flow cytometry | 100 mM [14] |
Source of the Controversy: EtBr as a Mutagen
The assumption that EtBr is dangerous to humans stems from the fact that it mutates DNA by intercalating it, which could result in genetic mutations owing to effects on subsequent DNA replication, transcription, and translation processes. [1,10]
Although in vitro tests on various cultured cell lines and embryo systems have indicated that the use of EtBr can lead to frame-shift mutations, chromosomal recombination, arrested cell division, and developmental problems, there is no conclusive evidence to demonstrate these effects in humans. [10]
This information is summarized in an excellent report on EtBr from the National Toxicology Program.
These in vitro tests, which comprise the entire body of evidence on which the EtBr controversy is built, don’t provide any evidence that EtBr can exert a genotoxic effect in anything more complicated than a single cell or an unprotected embryo.
In fact, there is no direct evidence implicating EtBr as a carcinogen in any animal. As we mentioned at the beginning of this article, EtBr has been used for over 60 years as a treatment for African sleeping sickness in cattle.
For this purpose, EtBr is administered via subcutaneous or intramuscular injection with no reported increase in the incidence of tumor formation or birth defects in the treated cattle. [3,5,10]
This suggests that EtBr is far less genotoxic to animal systems than is presumed from the in vitro data. If it were as genotoxic as presumed, would we not expect to see mutant cows roaming the African continent?
The standard non-toxic dose of EtBr is 1 mg/kg [3,5] in cattle, which equates to a blood concentration of approximately 0.075 μg/mL 24 hours after administration. [5]
In comparison, the standard concentration used in molecular biology (around 1 mg/L), is low. Microbiologist Rosie Redfield [15] puts this into perspective:
“A 50 kg researcher would need to drink 50,000 liters of gel-staining solution to get even the non-toxic dose used in cattle.”
To put this further into context, EtBr is less toxic than salicylates (e.g., aspirin) and caffeine, which cause severe toxicity at serum concentrations of >750 mg/L [16] and >80 mg/L, [17,18] respectively.
Just think about how commonly these two are consumed.
From this, the risks posed to a scientist handling a standard solution (e.g. containing 0.5 μg/mL EtBr, a typical concentration for agarose gel electrophoresis [7,13]), with a gloved hand (remember the cattle are injected with the stuff) are put into perspective.
Disposal of EtBr Waste
Improper disposal of lab waste can have serious consequences for the environment, biodiversity, and public health. [19]
While there is no direct evidence to suggest that EtBr is toxic to humans at the typical concentrations used in the lab (Table 1), its effects on the environment are not fully understood. [19]
Therefore, attempts should be made to dispose of EtBr waste in a safe and responsible way. Some common disposal methods are described in Table 2.
Table 2. Methods of EtBr disposal
Disposal Method | Description | Notes |
Incineration | Agarose gels containing EtBr are often disposed of in hazardous waste bins, which, once full, are permanently closed, and incinerated at commercial waste facilities | Incineration allows for the complete destruction of most organic waste and reduces the volume of waste that must later be disposed of in landfill. However, it is expensive and requires large amounts of fuel to generate the necessary temperatures [20] Moreover, there are general concerns surrounding waste incineration and air, surface- and ground-water pollution [21] |
Activated charcoal | EtBr can be filtered from buffer solutions through commercially available filters or destaining bags containing activated charcoal. Following filtration or overnight incubation, respectively, the filtrate can be disposed of down the sink. Activated charcoal filters have been shown to bind up to 174 μg of EtBr depending on the type of solution [22] Once they have reached the end of their lifetimes, these filters or destaining bags can be disposed of as hazardous waste and incinerated | Some argue that it is perhaps counterintuitive to make a potentially dangerous chemical more concentrated by absorbing it onto charcoal However, this is a simple, effective, and relatively cheap method of EtBr disposal [22] |
Hypophosphorous acid, sodium nitrite, and sodium bicarbonate | EtBr-containing solutions can be diluted to a final concentration of 0.5 μg/mL. After incubation for 20 hours with 5% (v/v) hypophosphorous acid and 0.5 M sodium nitrate (with agitation), the mixture can be neutralized with sodium bicarbonate and subsequently discarded in the sink. This process should be performed in a fume hood [23] | This method of EtBr removal generates non-mutagenic reaction mixtures and degrades >99% of EtBr [23] |
AmberliteTM XAD-16TM | EtBr can be effectively removed using AmberliteTM XAD-16TM resin [23] | This is a relatively cheap and effective method of EtBr decontamination [23] |
In decades gone by, it was common practice to add sodium hypochlorite (i.e. bleach) to EtBr solutions before disposal down the sink. [24]
However, Lunn and Sansone (1987) showed that the combination of bleach and EtBr actually produces more toxic reaction products. [23,25]
In addition, bleach is an inefficient method of EtBr decontamination in this context because it leaves behind ~20% of its original mutagenicity. [26]
The moral of the story—don’t use bleach to clean up EtBr spills or as a method of disposal and think carefully about how you are disposing of your EtBr solutions.
Your departmental safety officer is a good person to consult to ensure you are abiding by your institute’s regulations.
More novel and potentially promising forms of EtBr degradation include phytoremediation (i.e. removal of pollutants by plants) [27,28], bioremediation by bacteria, [29] and phycoremediation by microalgae. [30]
While these three methods are interesting and exciting, further research is required to develop them into viable methods of EtBr disposal in the future.
Are the “Safer” Alternatives Actually Safer?
A real concern is that the irrational and ill-informed fear of EtBr drives us to solutions that are more dangerous than EtBr itself.
This could take the form of alternatives that are explicitly marketed as safer substitutes for EtBr (Table 3). But are they actually safer? Not always.
Thermo Fisher Scientific claimed that their SYBR® Safe DNA stain, a DNA minor groove binder instead of an intercalator, [31] was non-mutagenic, non-genotoxic, and non-hazardous. [32,33]
But as Rosie Redfield points out in her excellent article, SYBR Safe has higher acute toxicity in mice than EtBr. [15] Beware of clever marketing and don’t believe everything you read.
Table 3. Some examples of alternative nucleic acid dyes
Alternative to EtBr | Notes |
DiamondTM Nucleic Acid Dye (Promega) | Can be visualized using a UV or blue-light transilluminator [34] Can also be used for touch DNA localization [35] While SYBR Green can inhibit PCR, the DiamondTM Nucleic Acid Dye can also be used as an alternative for qPCR detection [36] |
SYBR® Safe & SYBR® Green (II) (Thermo Fisher Scientific) | Both can be visualized using a UV or blue-light transilluminator and are not mutagenic unlike SYBR Green (I) [32,37,38] Can be disposed of using standard lab procedures |
RedSafeTM (Chembio) | Claims to be “non-carcinogenic but may cause skin and eye irritations”. Can be disposed of in accordance with standard lab procedures [39] |
GelRedTM & GelGreenTM (Biotium) | Biotium claims that because both dyes have a greater mass than EtBr, to improve safety, they are unable to cross cell membranes, making them non-toxic and non-mutagenic GelRedTM is an intercalator and Biotium suggests that it is more sensitive than EtBr |
Make an Informed Decision
To reiterate, our take-home message on this would be to critically consider the hype and myths you have read about EtBr.
Read the articles I have cited, do your own research, arm yourself with the published data. Then make your own informed decision on how to handle EtBr or what alternatives to use, a decision based on fact…not hysteria.
As always, your comments are welcome!
Originally published September 26, 2007. Reviewed and updated by Zandile Nare December 2021.
References
- Waring MJ (1965) Complex formation between ethidium bromide and nucleic acids. J Mol Biol 13(1):269–82.
- Olmsted J et al. (1977) Mechanism of ethidium bromide fluorescence enhancement on binding to nucleic acids. Biochemistry 16(16):3647–54.
- Giordani F et al. (2016) The animal trypanosomiases and their chemotherapy: a review. Parasitology 143(14).
- Kinabo L (1993) Pharmacology of existing drugs for animal trypanosomiasis. Acta Trop 54(3–4):169–83.
- Chowdhury AR et al. (2010). The killing of African trypanosomes by ethidium bromide. PLoS Pathogens 6(12): e1001226
- Stevenson P et al. (1995) Comparison of isometamidium chloride and homidium bromide as prophylactic drugs for trypanosomiasis in cattle at Nguruman, Kenya. Acta Trop 59(2):77–84.
- Voytas D (1992) Agarose gel electrophoresis. Curr Prot Immunol 2(1).
- Chaudhuri K. Recombinant DNA Technology. The Energy and Resources Institute (TERI), 2013.
- Sayas E et al. (2015) Toxicity, mutagenicity and transport in Saccharomyces cerevisiae of three popular DNA intercalating fluorescent dyes. Yeast 32(9):595–606.
- The Myth of Ethidium Bromide. Available at: https://www.science.org/content/blog-post/myth-ethidium-bromide (accessed October 15, 2021).
- Schaffner-Barbero C et al. (2018) Pharmacological inhibition of the vacuolar ATPase in bloodstream-form Trypanosoma brucei rescues genetic knockdown of mitochondrial gene expression. Antimicrob Agents Chemother 62(9):e02268-17.
- Miskinyte M et al. (2020) A novel high-content phenotypic screen to identify inhibitors of mitochondrial DNA maintenance in trypanosomes. bioRxiv 2020.07.16.207886.
- Lee PY et al. (2012) Agarose gel glectrophoresis for the separation of DNA fragments. JoVE 62:3923.
- Whittle EE et al. (2019) Flow cytometric analysis of efflux by dye accumulation. Front Microbiol 10:2319.
- Redfield R. (2006) Heresy about Ethidium Bromide (accessed October 17, 2021).
- Dargan PI (2002) An evidence based flowchart to guide the management of acute salicylate (aspirin) overdose. Emerg Med J 19(3):206–9.
- Beauchamp G et al. (2017) Caffeine toxicity: A brief review and update. Clin Ped Emerg Med 18(3):197–202.
- Musgrave IF et al. (2016) Caffeine toxicity in forensic practice: possible effects and under-appreciated sources. Forensic Sci Med Pathol 12(3):299–303.
- Singh S et al. (2018) Ethidium bromide: Is a stain turning into a pollutant? A synthesis on its status, waste management, monitoring challenges and ecological risks to the environment. IJRAR 5(4):226–33.
- Laboratory NRC (US) (2011) C on PP in the Management of Waste. National Academies Press (US).
- Incineration NRC (US) (2000) C on HE of Waste Incineration Overview. National Academies Press (US).
- Menozzi FD et al. (1990) Absorption method for rapid decontamination of solutions of ethidium bromide and propidium iodide. Chromatographia 29(3–4):167–9.
- Lunn G et al. (1987) Ethidium bromide: destruction and decontamination of solutions. Analyt Biochem 162(2):453–8.
- Karcher SJ (1995) Recombinant DNA Cloning. In Karcher SJ, (ed.), Molecular Biology. San Diego: Academic Press, pp. 45–134.
- Cohen BL (1987) Ethidium bromide safety. Trends Genet 3:308.
- Quillardet P et al. (1988) Ethidium bromide and safety — readers suggest alternative solutions. Trends Genet 4(4):89–90.
- Amirijavid S et al. (2015) Phytoremediation of ethidium bromide by tomato and alfalfa plants. Int J Plant Biol Res 3(1):1029.
- Uera RB et al. (2007) Phytoremediation potentials of selected tropical plants for ethidium bromide. Env Sci Poll Res Int 14(7):505–9.
- Sukhumungoon P et al. Biodegradation of ethidium bromide by Bacillus thuringiensis isolated from soil. Afr J Microbiol Res 7(6):471–6.
- de Almeida HC et al. (2020) Phycoremediation potential of microalgae species for ethidium bromide removal from aqueous media. Int J Phytoremed 22(11):1168–74.
- Haines AM et al. (2015) Properties of nucleic acid staining dyes used in gel electrophoresis. Electrophoresis 36(6):941–4.
- Martineau C et al. (2008) Development of a SYBR safe technique for the sensitive detection of DNA in cesium chloride density gradients for stable isotope probing assays. J Microbiol Methods 73(2):199–202.
- Evenson WE et al. (2012) 1H and 13C NMR Assignments for the Cyanine Dyes SYBR Safe and Thiazole Orange. J Org Chem 77(23):10967–71.
- Cronan L. A Diamond in the Rough: New Applications of Diamond Nucleic Acid Dye. Promega Connections. 2019 (accessed November 12, 2021).
- Tonkrongjun P, et al. (2017) Touch DNA localization and direct PCR: An improved workflow for STR typing from improvise explosive devices. For Sci Int Genet Suppl Series 6:e610–2.
- Optimization of Diamond Nucleic Acid Dye for quantitative PCR | BioTechniques (accessed November 12, 2021).
- Singer VL et al. (1999) Comparison of SYBR® Green I nucleic acid gel stain mutagenicity and ethidium bromide mutagenicity in the Salmonella/mammalian microsome reverse mutation assay (Ames test). Mut Res/Gene Toxicol Environ Mutagen 439(1):37–47.
- SYBR Safe Frequently Asked Questions – UK (accessed November 12, 2021).
- Khalili E et al. (2015) Rapid fluorometric quantification of bacterial cells using Redsafe nucleic acid stain. Iran J Microbiol 7(6):319–23.