Metabolic tracing is one of the most powerful tools to study metabolic pathway activity. As a biologist, entering the world of analytical chemistry techniques can be intimidating.
That’s why we’re providing a beginner’s guide that will help make metabolic tracing understandable, approachable, and applicable to everyday research questions.
What Is Metabolic Tracing and Why Should I Care?
Metabolic pathways include all the chemical reactions in an organism that sustain biological function, from DNA replication to post-translational modifications.
Specifically, metabolic pathways consist of linked chemical reactions that convert a given molecule into a series of intermediates before producing a product that will eventually be excreted.
In biological research, techniques that allow us to follow the fate of molecules through these reactions can provide powerful biological insights, such as:
- Glutamine use changes as cells progress from non-malignant to malignant. [1]
- An intermediate in amino acid catabolism drives endothelial cell fatty acid uptake. [2]
- The nucleus and cytosol use different carbon sources for protein modifications. [3]
One such technique is metabolic tracing, in which a molecule of interest is given a specific label (more on these later) and thus made “trackable” via a variety of methods, including:
- Detecting a radioactive isotope label using a scintillation analyzer.
- Detecting a stable isotope label using mass spectrometry or NMR.
Metabolic tracing has enabled the discovery of new fundamental biology with important implications for human health.
For example, labeling fructose showed that the small intestine, rather than the liver, is actually the main site of fructose clearance and helps shield the liver from the potentially toxic effects of fructose. [4]
Or that oral nicotinamide ribose, a NAD precursor and commonly consumed supplement, actually contributes to NAD synthesis via the gut microbiome. [5]
What Can Metabolic Tracing Help Me Learn?
Metabolic tracing can help you answer all sorts of questions you might have about your biological system, including (but not limited to!):
- Which nutrients provide fuel for a given cell function, such as protein acetylation.
- How environmental perturbations, such as hypoxia, change nutrient use by the cell or organism.
- Whether changes in nutrient consumption have functional consequences, such as cell proliferative abilities.
- Why certain cell types might prefer one nutrient over another, i.e., what they are using these preferred nutrients to do.
- Where nutrients are being consumed and how this might change under pathologic conditions.
What Can Metabolic Tracing Tell Me That Metabolomics Can’t?
The boom of ‘omics-based approaches has given rise to thousands of metabolomics datasets. [6]
The appeal of metabolomics is understandable- information about thousands of different metabolites, all from a single experiment. [7]
Often, however, following up on interesting questions generated from metabolomics data becomes challenging.
The biggest problem when interpreting metabolomics data is metabolomics offers a static picture of a dynamic system. [8] Metabolites are constantly being produced and consumed by an incredibly complex network of enzymatic reactions.
If a given metabolite appears up- or down-regulated in metabolomics data, it is impossible to tell which process is driving the change—metabolite production or consumption.
For example, let’s say you notice plasma glucose levels are increased in a treatment group compared to a control group. Are the increased glucose levels due to increased glucose production or decreased glucose consumption?
Enter metabolic tracing! Metabolic tracing allows us to answer questions about where a given metabolite comes from (production) and where it’s going (consumption).
By labeling individual atoms in a given metabolite of interest, we can track the fate of these atoms over time and through pathways, giving us a dynamic understanding of a dynamic system.
Metabolomics data alone provides an incomplete story, and metabolic tracing helps fill this gap by measuring pathway activity.
Isotopes. What Are They and What Makes Them Stable?
So now you’re convinced and want to do some metabolic tracing. To set up, execute, and interpret your experiments, you need to know what isotopes are.
Simply put, isotopes are different forms of the same chemical element.
Atoms consist of protons, neutrons, and electrons. Protons and neutrons comprise the nucleus of an atom, while the electrons balance the positive charge of the protons and orbit the nucleus. Neutrons carry no charge.
The isotopes of a given element have the same number of protons but a different number of neutrons, so they have different masses.
For example, hydrogen has 3 naturally occurring isotopes, with 0, 1, and 2 neutrons (Figure 1).
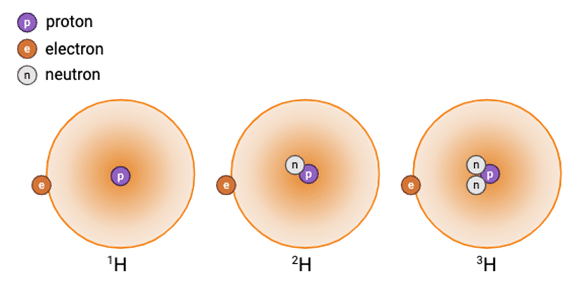
Figure 1. The 3 naturally occurring isotopes of hydrogen. Left-to-right: hydrogen, deuterium, and tritium. (Image credit: Biorender.com).
You might have heard isotopes or isotope tracers referred to as “stable,” which simply means non-radioactive isotopes.
Conversely, radioactive isotopes are unstable. They decay to a stable isotope by emitting radiation in the form of either:
- Alpha radiation (a cluster of two protons and two neutrons).
- Beta radiation (either an electron or a positron).
- Gamma radiation (a high-energy, short-wavelength electromagnetic wave).
While radioactive (or “hot”) isotope tracers used to be favored for their high signal-to-noise ratio, stable isotopes are now broadly used due to their excellent safety profile.
How Are Stable Isotopes Used in Metabolic Tracing?
Metabolic isotope tracers are physiologically indistinguishable from endogenous metabolites (metabolites naturally produced by the organism) but detectable via mass spectrometry.
While many different atoms can be labeled with isotope tracers, the most common in metabolic applications are carbon, nitrogen, and hydrogen.
For example, the carbons in glucose can be the “13C” isotope rather than “12C”, making them slightly heavier.
First, we must introduce our metabolic isotope tracer into our biological system. This can be done by:
In vivo
- Intravenous infusion.
- Injection (ex. Intraperitoneal).
- Oral delivery (gavage, food, water).
In vitro
- Perfusion (ex. Langendorff technique).
- Incubation (cell culture media, buffers, etc.)
After introducing our tracer, we can track our labeled atom as it undergoes metabolic enzyme conversions.
Sticking with our example of glucose, we can check and see if our labeled carbons eventually made their way into lactate, or possibly lipid stores (Figure 2).
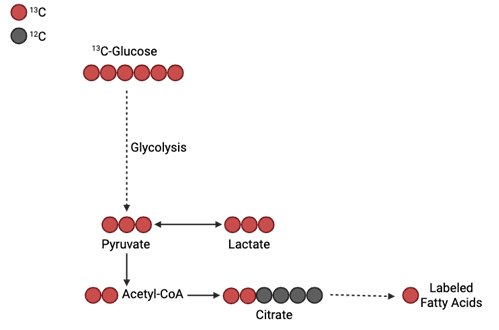
Figure 2. Example of tracking labeled carbons from a 13C-Glucose tracer into lactate and fatty acids (Image credit: Biorender.com).
Crucial Considerations for Designing a Metabolic Tracing Experiment
Which Atom Do I Want to Follow
Make sure the labeled atom you use participates in your metabolic pathways of interest. For example, you wouldn’t want to label a carbon in a molecule that will get “lost” as CO2 before it reaches your downstream metabolite of interest.
How Sensitive Are My Detection Capabilities?
Knowing the sensitivity range of your detection method will help guide experimental design questions such as the concentration and rate of isotope delivery.
Should I Worry About Disturbing Endogenous Physiology?
If you’re isotope delivery is too low, you won’t be able to detect it (see “How Sensitive Are My Detection Capabilities”), but if you aim too high you risk disturbing endogenous metabolism, hence the importance of pilot experiments to balance these two considerations.
What are the Kinetics of My Biological Processes of Interest?
Metabolic pathways can operate on vastly different timescales and thus require varying lengths of isotope tracer exposure to accumulate detectable products. [9] For example, detecting labels in synthesized protein would require a much longer experiment than detecting glycolysis-produced lactate.
Am I Interested in the Rate of a Pathway and Which Nutrients Fuel a Pathway
In other words, are you interested in what fuel the engine is burning, or how fast it’s burning it. Answering these questions requires different tracing strategies.
Have I Identified Confounding Variables?
Like every experiment, it’s important to consider factors that may convolute the interpretation of your labeling results. For example, Carbon-labeled glucose may be converted to lactate before entering oxidative pathways, making you unable to distinguish whether your labeled carbons came directly from glucose or glucose-derived lactate. [10]
Summary
When carefully planned, metabolic tracing experiments enable the direct tracking of atoms through metabolic pathways via detecting differences in mass.
This tracking system helps answer questions about highly dynamic biological systems that other techniques, such as metabolomics, cannot.
Thinking about the kinetics, sensitivity, and properties of your biological system of interest can help guide your experimental design.
Once you’ve dipped your toes into metabolic tracing, you can start thinking about more advanced applications, such as imaging mass spec. [11]
If you have experience with metabolic tracing, share in the comments section your best tips for successful experimental design, or your favorite resources to guide interpreting tracing data!
References
1. Gonsalves WI, Jang JS, Jessen E, Hitosugi T, Evans LA, Jevremovic D, Nair KS et al. (2020). In vivo assessment of glutamine anaplerosis into the TCA cycle in human pre-malignant and malignant clonal plasma cells. Cancer metab. 8:1-17
2. Jang C, Oh SF, Wada S, Rowe GC, Liu L, Chan MC, Arany Z et al. (2016). A branched-chain amino acid metabolite drives vascular fatty acid transport and causes insulin resistance. Nat med. 22(4):421–26
3. Trefely S, Huber K, Liu J, Noji M, Stransky S, Singh J, Snyder NW (2022). Quantitative subcellular acyl-CoA analysis reveals distinct nuclear metabolism and isoleucine-dependent histone propionylation. Mol cell. 82(2):447–62
4. Jang C, Hui S, Lu W, Cowan AJ, Morscher RJ, Lee G, Liu W, Tesz GJ, Birnbaum MJ, Rabinowitz JD (2018). The Small Intestine Converts Dietary Fructose into Glucose and Organic Acids. Cell metab. 27(2):351–61.e3
5. Chellappa, K, McReynolds MR, Lu W, Zeng X, Makarov M, Hayat F, Baur JA et al. (2022). NAD precursors cycle between host tissues and the gut microbiome. Cell metab. 34(12):1947–59
6. Learn-omics! What is this “Omics” I Keep Stumbling Upon? Available at: https://bitesizebio.com/24629/learn-omics-what-is-that-omics-i-keep-stumbling-upon Accessed June 15, 2024
7. The Importance of Metabolomics Research. Available at: https://bitesizebio.com/31115/importance-metabolomics Accessed June 15, 2024
8. Jang C, Chen L, Rabinowitz JD. (2018). Metabolomics and Isotope Tracing. Cell 173(4):822–37
9. Bartman CR, TeSlaa T, Rabinowitz JD. (2021). Quantitative flux analysis in mammals. Nat metab. 3(7):896–908
10. Hui S, Ghergurovich J M, Morscher RJ, Jang C, Teng X, Lu W, Esparza, LA, Reya T, Zhan L, Yanxiang Guo J, White E, Rabinowitz JD. (2017). Glucose feeds the TCA cycle via circulating lactate. Nature. 551(7678):115–18
11. How to Choose Your MALDI (Soul) Matrix. Available at: https://bitesizebio.com/27800/how-to-choose-your-maldi-soul-matrix Accessed June 15, 2024