Two Photon Confocal Microscopy: What it is and How to Use it to Your Advantage
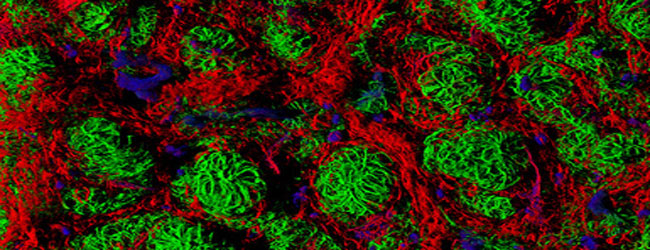
“A two photon microscope has higher sensitivity than a normal confocal microscope, because it uses two photos instead of one!” Yes, I can bear witness that this phrase has actually been uttered, and it was not by an undergraduate student.
No exception to the rule
The condensation of various levels of misunderstandings in this statement is phenomenal- one would be hard pressed to find more mistakes in such a short sentence! Not knowing how and why a microscope does what it does, is not just a gap in general knowledge. It can lead to lost opportunities for data acquisition- or to misinterpretation of available data. Consequently, it’s essential that research scientists involved in microscopy in some way or the other have a clear understanding of some of the principles behind the function of the imaging apparatus they are using. Two-photon microscopy is no exception to this rule.
Two into one
In 1931, theoretical physicist Maria Goeppert-Mayer (who later received the Nobel Prize) described the two-photon absorption phenomenon in her doctoral dissertation. She described the simultaneous absorption of two photons of identical or different frequencies, resulting in the excitation of a molecule from one state (usually the ground state) to a higher energy electronic state. Of course, this energy level shift is what happens in one-photon excitation (which is what we use when we excite a fluorophore in normal fluorescence microscopy- see this article for an introduction to fluorescence). However, in the two-photon case, the difference between the lower and upper states of the molecule is equal to the sum of the energies of the two photons, not of a single photon. For this to happen, the absorption of the two photons must be simultaneous.
Three or more
Two-photon absorption is several orders of magnitude weaker than one-photon absorption, as it depends on the probability of two photons being absorbed at the same time. Unlike one-photon absorption it is a nonlinear process, and the strength of absorption depends on the square of the light intensity. If the excitation is strong enough, three-photon and multi-photon excitation is also possible.
From theory to image
The effect was demonstrated in practice by Kaiser and Garret in 1963. The prospect of using the phenomenon for microscopy was first introduced in 1978 by Sheppard and Kompfner, and the first practical demonstration took place in 1990, when Denk and colleagues generated two-photon images of optical sections through chromosomes of live cultured pig kidney cells, stained with a viable DNA stain.
Intrinsically confocal
This technique is intrinsically confocal: all excitation happens at the focal plane, and all emission comes from the focal plane. More specifically, two-photon fluorescence occurs only at the microscope focal volume- this is the ‘in focus’ volume of a sample which is detected with the confocal microscope. Within the focal volume, the photon density is high- the probability of two-photon excitation occurrence drops exponentially with decreasing intensity outside the focal volume, with 80% of the total excitation happening within this volume. Subsequently, there is no need for pinhole apertures to reject the out-of-focus signal, as such signal practically doesn’t exist. A typical two-photon excitation point spread function has a full width at half-maximum of 0.3 mm in the radial direction and 0.9 mm in the axial direction when 960 nm excitation light is used, through an objective with a numerical aperture of 1.25.
Advantages
There are a number of advantages which two-photon excitation offers (compared to the usual one-photon technique):
1) Near-infrared radiation used in two-photon excitation suffers from significantly less absorption in biological specimens than UV or blue-green light, making the technique more appropriate for imaging thick specimens. The gradual loss in intensity (or ‘attenuation’) of excitation light from scattering is also reduced, as scattering decreases with decreasing excitation frequency.
2) Confocal microscopy achieves confocality by using the emission pinhole aperture to reject out-of-focus light. However, inside thick specimens, scattering of the fluorescent photons is inevitable, resulting in significant loss of photons at the confocal pinhole. Two-photon microscopy limits the excitation volume, requiring no pinhole aperture, thus minimizing signal loss.
3) Compared with confocal microscopy operating in higher frequencies (longer excitation wavelengths), two-photon microscopy induces less photobleaching and photodamage. A confocal microscope illuminates the specimen with a double inverted cone of light, with photobleaching and photodamage occurring in all this volume. A two-photon microscope causes photodamage limited to a sub-femtolitre volume at the focal point. The reduction in the photodamage volume results in a significant increase in viability of biological specimens.
4) A wider gap between excitation and emission makes it easier to reject excitation light, with minimal loss of emission photons.
5) The flip side of the fact that photodamage takes place only in a very small volume, is the inherent capacity to cause photochemical reactions, for example chemical photoactivation, or to be used for activation of optogenetic ‘switches’, in targeted sub-femtoliter volumes with high localization precision.
Disadvantages
Obviously, there is no such thing as a free lunch, and two-photon microscopy does have its inherent disadvantages. Remember Abbe’s law? The resolution of a microscope system is inversely proportional to the wavelength of light used. So, for a given fluorophore, as two-photon excitation uses approximately twice the one-photon wavelength, the result will be approximately half the resolution. But you need not despair. Two-photon STED (STED is a superresolution technique) has already been described!
Understandably, two-photon microscopy has become especially popular with researchers wanting to observe cells in tissue cultures (for example, in organotypic brain slice cultures, which can be around 300 µm thick) or even peek into the living brains of anaesthetized animals. How can this be done? Through a window! That’s right, a cranial window. A small piece of bone is replaced with a coverglass, which is sealed permanently in place using dental acrylic, thus creating an imaging window with a large field of view, which can be visited repeatedly. A somewhat less Frankestein-ish technique involves thinning of the skull and visualization through it. These techniques give us the previously unimaginable capacity to visualize living neurons in the intact, living cortex.
Under optimal conditions, imaging can be extended to several hundred micrometers depth, typically 6-fold deeper than confocal microscopy, for a given sample and fluorophore. Imaging of mouse cortical vasculature has been achieved in vivo with a record imaging depth of 1.6 mm.
The outstanding properties of two-photon imaging have started to find applications in the clinic, mostly for cancer cell imaging, including detection in microendoscopic and dermatology investigations.
1 Comments
Leave a Comment
You must be logged in to post a comment.
Under ‘Advantages’ item 3, you state “operating in higher frequencies (longer excitation wavelengths)”
Shouldn’t that be *shorter* excitation wavelengths?